Most of us expect for lights to turn on with the push of the button without giving any thought to how much our everyday lives depend on energy. Energy is not only used to power the electric devices we use, to heat our homes and to fuel the cars we drive, but also to manufacture and ship all the stuff we have around us. The amount of energy everyone in the developed world uses today was historically available only to kings. This is possible thanks to the enormous energy systems in our countries.
In the WISE project, we are looking at the resilience of complex social and technological systems. How for example can widespread energy systems survive extreme and unexpected events and recover afterwards? While many of the traditional threats to energy systems (primarily geopolitical risks, extreme weather events and technical failures) are well known, there are many important threats with little consideration given so far.
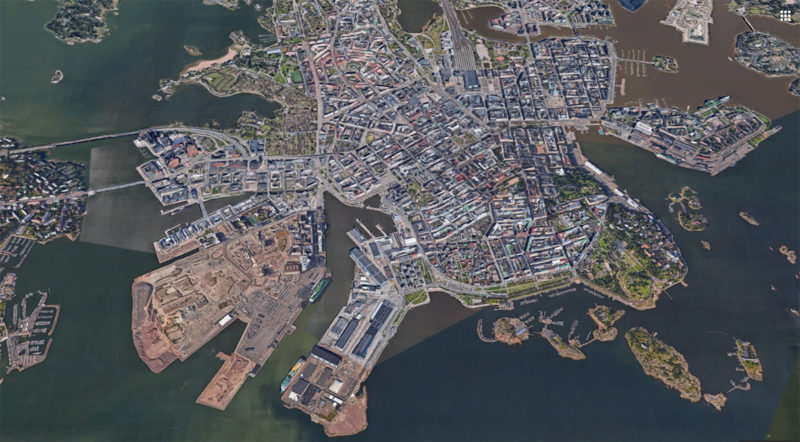
Renewable, distributed and digital – all good, right?
Renewable energy (RE) sources allow decarbonization of energy systems, which is urgently needed to mitigate major and potentially irreversible risks of climate change. However, shifting to renewable energy changes energy systems in various ways. Instead of fuel stocks, production flows are a main concern, and they are highly variable due to changing environmental conditions. The system will also be more decentralized. At the same time, monitoring and control of energy systems is being rapidly digitalized.
All these changes have the potential to increase the resilience of energy systems in the face of traditional threats. In fact, security considerations are used to promote development and adoption of renewable energy, sometimes more successfully than promotion based on environmental grounds. But as with all change, this will also present new risks. These four key examples call for caution in the energy transition.
Climate impacts to RE: Energy production from renewable sources inherently depends on variable weather conditions. As climate change progresses, current weather patterns are subject to change. This is a concern, as power producers need to ensure the economic payback of a plant during its operational lifetime. With increased proportion of renewable energy, unexpected decrease in production may become a capacity adequacy concern for the whole system. Even though existing studies project the changes to be small and context-dependent, results are subject to significant uncertainties.
Collapse of petro-states: For countries dependent on fossil fuel imports, this transition brings significant economic and security benefits. In the long run, even major exporting countries may benefit as they avoid “the resource curse” of oversized extractive sectors. But in the short term, the transition creates a lot of economic and political pressure. If pressures appear rapidly and are poorly managed, these states could collapse altogether. The low oil prices in 1980s are considered an important factor in decline and eventual collapse of the Soviet Union. Political and social instability of such events could spill over presenting major geopolitical risks.
Cybersecurity of energy systems: Digital systems in energy infrastructure control more and more critical operations, connect a plethora of devices, are accessed by an increasing number of people, use huge amounts of data and a variety of software solutions. This increases the potential to disturb system operation and damage infrastructure. Especially as connected devices are more widely distributed, high-level security options are not available in places like the homes of end-users. Numerous digital security solultions are developed for data networks, but they are often not applicable for fundamentally different energy systems. Increasing number of users heightens the possibility of careless use, and diversifying software solutions increase potential of security gaps. Cyber-attacks can be used alongside other means such as physical attacks and energy system operation personnel coercion. While cyber-security is a widely acknowledged issue, industry remains slow in taking appropriate measures.
Platforms: Recent developments in the field of smart grids have enabled new ways of controlling energy and information flows. Subsequently, digital platforms and algorithms are being developed to record and steer energy flows between end-use loads, power production, and storage units. Platforms also enable crowdfunded investments into renewable energy production. Digital platforms can promote decarbonization and enhance the system resilience. On the other hand, the same platforms can introduce new and complex dynamics with undesirable consequences. Simplicity and cost gains may be made at the expense of crucial social functions or public goods. Barriers against new entrants may emerge, resulting in market monopolization. Strain on the grid may increase. The resilience implications of these and other examples are often indirect and therefore not clear. Even benefits from the decentralized physical systems promoted by the platforms are not obvious, as the actual number of platforms themselves (judging from the developments in social networks) could be very small. Hacking a very large platform could have more damaging implications than even hacking a major component in a highly centralized power system.
How bad could it be?
Not all relevant threats to energy systems are known. Some unknown events, so called black swans, have the potential to cause enormous damage. But how can anyone prepare against the unknown? One way to do it is to consider worst-case scenarios irrespective of their likelihood. Investigating highly unlikely scenarios like demon invasion can perhaps provide valuable real-world insights, but examples of more likely threats with potentially catastrophic consequences are easy to find.
Cascading failures: These refer to sequential power system component failures caused by another component failure. Resulting damages could be very large, including system-wide blackouts, even when the initial disturbance is relatively weak. Cascading failures appear when system components are highly interdependent, which is not always visible due to overall system complexity. Duplication of system components can mitigate the effects of interdependence but cannot eliminate them.
Cascading failures within power systems have been studied for a long time, but risks continue to increase due to wider geographical interconnection. Thus the worst-case scenario is no longer a countrywide but a continent-wide blackout. With increasing cross-sectorial integration due to the digitalization of energy systems and the electrification of heating and transport, cascading failures across multiple sectors become more likely. From resilience perspective, cross-sectorial integration removes crucial “firebreaks” that allow isolation and containment of threats. Therefore, designing additional firebreaks could be important to avoid catastrophic all-encompassing failures.
Multiple contingences in a short time period: Disturbances affect the capability of energy systems to supply energy as well as to withstand such events in the future, their resilience capacity. Even when a system withstands the disturbance it was designed for, its capability to withstand another but not necessarily the same type of disturbance could be greatly reduced for some time. Multiple disturbances appearing in a short time period can cause damage that is much more significant than from events with longer time intervals between them. While the occurrence of multiple contingencies in a short period is unlikely, it is not impossible. Linkages between different contingencies would greatly increase the likelihood of such events. For example, a poorly maintained component is more likely to fail in a grid under stress from environmental conditions or due to failure of another component than during normal operation. Another example could be cyber-attacks performed strategically during or slightly after an extreme weather event.
Humans as part of energy system: Large numbers of people are working in planning, operating and maintaining energy systems. A lot of these jobs require specific qualifications limiting the pace of replacement, which could be an issue in case of severe epidemics or military conflicts. Other major human related risks include intentional damage done by insiders in an institution, poor management of security credentials, and operational mistakes. This is extremely important with critical infrastructure, such as nuclear power plants.
Space weather: The sun releases charged particles whose direction, amount and energy can vary significantly. During so-called geomagnetic storms, the flow of charged particles can be strong enough to disturb the Earth’s magnetosphere temporarily. These storms can create strong magnetic fields inducing large currents in long conductors, like power transmission and communication lines. These currents in the power system can be dangerous to various system components with transformers being among the most vulnerable. Most generators are connected to the grid through transformers and thus would not be strongly affected by such currents. However, in case of transformer failure, a sudden loss of load would put the generator at risk of overheating. A number of geomagnetic storms have been recorded with largest of them taking place in 1859. A storm of such magnitude today could damage a large number of power system components globally. Repairing all damaged components may require much more spare parts than there are stocked in. Capabilities of manufacturing and delivery of new components themselves would be affected by the storm. Modern power systems may also not be able to operate without well-functioning communication systems even if physical system components are in order. Prolonged dysfunction of energy systems could harm other vital systems, like food production, that relay significantly on uninterrupted energy supply.
***
Decarbonization of energy systems is a vital component in moving societies towards zero-carbon and further towards net carbon-negative economies. Renewable energy, increasing electrification and the attendant decentralization and cross-sectorial integration of energy systems are key features of avoiding the hell of climate change. But as the devil is in the details, other gates of hell may open if such challenges to resilience are not taken seriously.